Cas no 71186-63-5 (2-(Allyloxy)-3-methoxyphenol)

2-(Allyloxy)-3-methoxyphenol structure
商品名:2-(Allyloxy)-3-methoxyphenol
CAS番号:71186-63-5
MF:C10H12O3
メガワット:180.20048
CID:1748201
2-(Allyloxy)-3-methoxyphenol 化学的及び物理的性質
名前と識別子
-
- Phenol, 3-methoxy-2-(2-propenyloxy)-
- 2-(Allyloxy)-3-methoxyphenol
-
計算された属性
- せいみつぶんしりょう: 180.07866
じっけんとくせい
- PSA: 38.69
2-(Allyloxy)-3-methoxyphenol 価格詳細 >>
エンタープライズ | No. | 商品名 | Cas No. | 清らかである | 仕様 | 価格 | 更新日時 | 問い合わせ |
---|---|---|---|---|---|---|---|---|
TRC | A262300-2.5g |
2-(Allyloxy)-3-methoxyphenol |
71186-63-5 | 2.5g |
$ 597.00 | 2023-04-19 | ||
TRC | A262300-10g |
2-(Allyloxy)-3-methoxyphenol |
71186-63-5 | 10g |
$ 2067.00 | 2023-04-19 | ||
TRC | A262300-1g |
2-(Allyloxy)-3-methoxyphenol |
71186-63-5 | 1g |
$ 253.00 | 2023-04-19 | ||
TRC | A262300-5g |
2-(Allyloxy)-3-methoxyphenol |
71186-63-5 | 5g |
$ 1166.00 | 2023-04-19 |
2-(Allyloxy)-3-methoxyphenol 関連文献
-
Sean W. Reilly,Charles Edwin Webster,T. Keith Hollis,Henry U. Valle Dalton Trans., 2016,45, 2823-2828
-
Xiao-Wei Zhang,Zu-Feng Xiao,Mei-Mei Wang,Yan-Jun Zhuang,Yan-Biao Kang Org. Biomol. Chem., 2016,14, 7275-7281
-
Krishna K. Pandey Dalton Trans., 2012,41, 3278-3286
-
Qiongqiong Wang,Liping Qiao,Min Zhou,Shan Wang,Shengrong Lou,Dandan Huang,Qian Wang,Shengao Jing,Hongli Wang,Changhong Chen,Cheng Huang Faraday Discuss., 2021,226, 112-137
-
Ruizhi Li,Na Liu,Bingqiang Li,Yinong Wang,Guolin Wu,Jianbiao Ma Polym. Chem., 2015,6, 3671-3684
71186-63-5 (2-(Allyloxy)-3-methoxyphenol) 関連製品
- 2640889-46-7(6-Cyclopropyl-5-fluoro-2-[4-(5-fluoropyrimidin-4-yl)piperazin-1-yl]-3,4-dihydropyrimidin-4-one)
- 2034569-13-4(N-2-(1-benzothiophen-3-yl)-2-(dimethylamino)ethyl-3,4-difluorobenzamide)
- 1368927-01-8(3-amino-2-(3,4-difluorophenyl)propan-1-ol)
- 172514-47-5(4-amino-2,6-dichlorobenzene-1-sulfonamide)
- 1807249-40-6(Methyl 2-cyano-3-trifluoromethoxy-5-(trifluoromethylthio)benzoate)
- 2248276-77-7(rac-1,3-dioxo-2,3-dihydro-1H-isoindol-2-yl (4R,6R)-2-oxo-6-phenylpiperidine-4-carboxylate)
- 1251613-28-1(4-(3,5-dimethyl-1-phenyl-1H-pyrazol-4-yl)-N-(thiophen-2-yl)methylpiperazine-1-carboxamide)
- 946234-91-9(ethyl 4-(4-methylphenyl)-3-(phenylsulfamoyl)thiophene-2-carboxylate)
- 2034353-56-3(methyl 3,4-diethoxy-5-{6-methyl-4-oxo-4H,5H-1,2,3triazolo1,5-apyrazine-3-amido}benzoate)
- 2010325-18-3(8-azabicyclo3.2.1octane-3-thiol)
推奨される供給者
上海贤鼎生物科技有限公司
ゴールドメンバー
中国のサプライヤー
大量
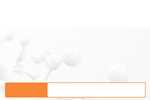
HUNAN CHEMFISH PHARMACEUTICAL CO.,LTD
ゴールドメンバー
中国のサプライヤー
大量
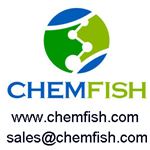
Shenzhen Jianxing Pharmaceutical Technology Co., Ltd.
ゴールドメンバー
中国のサプライヤー
試薬
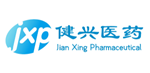
Nanjing Jubai Biopharm
ゴールドメンバー
中国のサプライヤー
大量
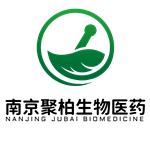
Jiangsu Xinsu New Materials Co., Ltd
ゴールドメンバー
中国のサプライヤー
大量
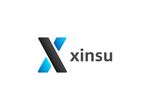