Cas no 2227906-19-4 ((2S)-2-(2-bromo-4-nitrophenyl)oxirane)
(2S)-2-(2-ブロモ-4-ニトロフェニル)オキシランは、高純度の光学活性エポキシド化合物であり、不斉合成における重要な中間体として利用されます。ブロモ基とニトロ基を有する芳香環にエポキシ環が結合した特異な構造を持ち、求核試薬との反応性に優れています。特に立体選択的反応において、高い位置選択性と立体特異性を発揮する点が特徴です。医薬品中間体や機能性材料の合成において、光学活性を保持したままの骨格構築が可能であり、有機合成化学の研究分野で有用性が認められています。高い反応性と立体化学的制御性を兼ね備えた化合物として知られています。
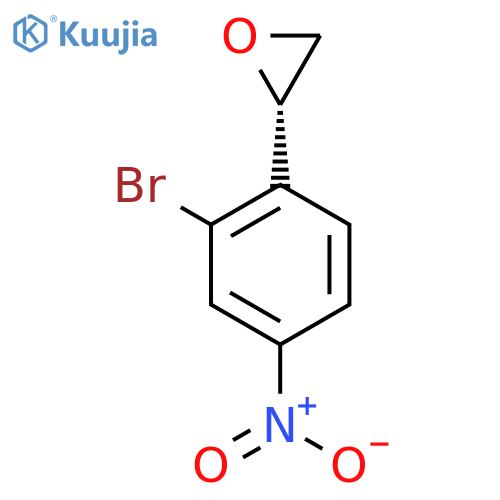
2227906-19-4 structure
商品名:(2S)-2-(2-bromo-4-nitrophenyl)oxirane
(2S)-2-(2-bromo-4-nitrophenyl)oxirane 化学的及び物理的性質
名前と識別子
-
- (2S)-2-(2-bromo-4-nitrophenyl)oxirane
- 2227906-19-4
- EN300-1927918
-
- インチ: 1S/C8H6BrNO3/c9-7-3-5(10(11)12)1-2-6(7)8-4-13-8/h1-3,8H,4H2/t8-/m1/s1
- InChIKey: YEQFIRRLIZSUFG-MRVPVSSYSA-N
- ほほえんだ: BrC1C=C(C=CC=1[C@H]1CO1)[N+](=O)[O-]
計算された属性
- せいみつぶんしりょう: 242.95311g/mol
- どういたいしつりょう: 242.95311g/mol
- 同位体原子数: 0
- 水素結合ドナー数: 0
- 水素結合受容体数: 3
- 重原子数: 13
- 回転可能化学結合数: 1
- 複雑さ: 218
- 共有結合ユニット数: 1
- 原子立体中心数の決定: 1
- 不確定原子立体中心数: 0
- 化学結合立体中心数の決定: 0
- 不確定化学結合立体中心数: 0
- トポロジー分子極性表面積: 58.4Ų
- 疎水性パラメータ計算基準値(XlogP): 1.9
(2S)-2-(2-bromo-4-nitrophenyl)oxirane 価格詳細 >>
エンタープライズ | No. | 商品名 | Cas No. | 清らかである | 仕様 | 価格 | 更新日時 | 問い合わせ |
---|---|---|---|---|---|---|---|---|
Enamine | EN300-1927918-0.25g |
(2S)-2-(2-bromo-4-nitrophenyl)oxirane |
2227906-19-4 | 0.25g |
$1432.0 | 2023-09-17 | ||
Enamine | EN300-1927918-10g |
(2S)-2-(2-bromo-4-nitrophenyl)oxirane |
2227906-19-4 | 10g |
$6697.0 | 2023-09-17 | ||
Enamine | EN300-1927918-0.05g |
(2S)-2-(2-bromo-4-nitrophenyl)oxirane |
2227906-19-4 | 0.05g |
$1308.0 | 2023-09-17 | ||
Enamine | EN300-1927918-2.5g |
(2S)-2-(2-bromo-4-nitrophenyl)oxirane |
2227906-19-4 | 2.5g |
$3051.0 | 2023-09-17 | ||
Enamine | EN300-1927918-0.5g |
(2S)-2-(2-bromo-4-nitrophenyl)oxirane |
2227906-19-4 | 0.5g |
$1495.0 | 2023-09-17 | ||
Enamine | EN300-1927918-0.1g |
(2S)-2-(2-bromo-4-nitrophenyl)oxirane |
2227906-19-4 | 0.1g |
$1371.0 | 2023-09-17 | ||
Enamine | EN300-1927918-1.0g |
(2S)-2-(2-bromo-4-nitrophenyl)oxirane |
2227906-19-4 | 1g |
$1557.0 | 2023-06-01 | ||
Enamine | EN300-1927918-10.0g |
(2S)-2-(2-bromo-4-nitrophenyl)oxirane |
2227906-19-4 | 10g |
$6697.0 | 2023-06-01 | ||
Enamine | EN300-1927918-5.0g |
(2S)-2-(2-bromo-4-nitrophenyl)oxirane |
2227906-19-4 | 5g |
$4517.0 | 2023-06-01 | ||
Enamine | EN300-1927918-5g |
(2S)-2-(2-bromo-4-nitrophenyl)oxirane |
2227906-19-4 | 5g |
$4517.0 | 2023-09-17 |
(2S)-2-(2-bromo-4-nitrophenyl)oxirane 関連文献
-
Stephen Caddick RSC Adv., 2013,3, 14975-14978
-
Tian Wen,Jian Zhang CrystEngComm, 2016,18, 218-221
-
Keiko Okuzumi,Noriyuki Hara,Hidehiro Uekusa,Yoshinori Fujimoto Org. Biomol. Chem., 2005,3, 1227-1232
-
Zhentan Lu,Lin Mei,Xinge Zhang,Yanan Wang,Yu Zhao,Chaoxing Li Polym. Chem., 2013,4, 5743-5750
2227906-19-4 ((2S)-2-(2-bromo-4-nitrophenyl)oxirane) 関連製品
- 2229526-53-6(5-(2-methyloxiran-2-yl)-1-benzofuran)
- 64869-63-2((1S,3R)-3-methylcyclohexan-1-amine)
- 1782356-84-6(7-Methyl-1,2-dihydroisoquinolin-3(4H)-one)
- 1864125-83-6(1-(5-amino-2-fluorophenyl)pentan-1-one)
- 2126178-06-9(tert-butyl N-[(1-benzyl-6-fluoro-1,2,3,4-tetrahydroquinolin-3-yl)methyl]carbamate)
- 2228237-62-3(4-1-(aminooxy)-2-methylpropan-2-yl-2-fluoro-N,N-dimethylaniline)
- 2229607-52-5(4-(3-bromoprop-1-en-2-yl)-2-methyl-1-nitrobenzene)
- 1396872-59-5(4-({1-(2-cyclopentylacetyl)piperidin-4-ylmethoxy}methyl)benzonitrile)
- 1361490-29-0(4,3',4',5'-Tetrachloro-2,3,5,6-tetrafluorobiphenyl)
- 1803777-04-9(Ethyl 2-cyano-3-hydroxy-4-(trifluoromethoxy)benzoate)
推奨される供給者
Hebei Ganmiao New material Technology Co., LTD
ゴールドメンバー
中国のサプライヤー
大量
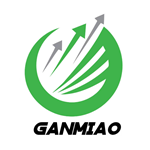
Nantong Boya Environmental Protection Technology Co., Ltd
ゴールドメンバー
中国のサプライヤー
大量
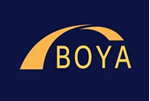
Nanjing jingzhu bio-technology Co., Ltd.
ゴールドメンバー
中国のサプライヤー
大量
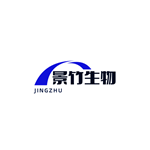
Amadis Chemical Company Limited
ゴールドメンバー
中国のサプライヤー
試薬
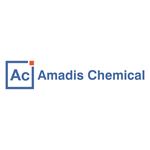
BIOOKE MICROELECTRONICS CO.,LTD
ゴールドメンバー
中国のサプライヤー
試薬
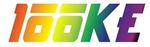