Cas no 2172460-31-8 (benzyl N-{3-2-(chlorosulfonyl)ethyloxetan-3-yl}carbamate)
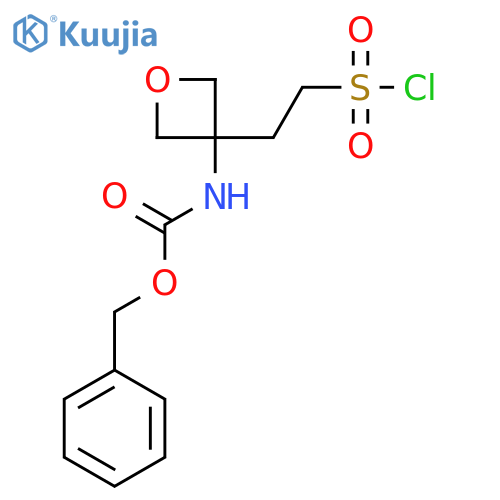
2172460-31-8 structure
商品名:benzyl N-{3-2-(chlorosulfonyl)ethyloxetan-3-yl}carbamate
benzyl N-{3-2-(chlorosulfonyl)ethyloxetan-3-yl}carbamate 化学的及び物理的性質
名前と識別子
-
- benzyl N-{3-2-(chlorosulfonyl)ethyloxetan-3-yl}carbamate
- 2172460-31-8
- EN300-1440620
- benzyl N-{3-[2-(chlorosulfonyl)ethyl]oxetan-3-yl}carbamate
-
- インチ: 1S/C13H16ClNO5S/c14-21(17,18)7-6-13(9-19-10-13)15-12(16)20-8-11-4-2-1-3-5-11/h1-5H,6-10H2,(H,15,16)
- InChIKey: LLDRDSXBPKJKDS-UHFFFAOYSA-N
- ほほえんだ: ClS(CCC1(COC1)NC(=O)OCC1C=CC=CC=1)(=O)=O
計算された属性
- せいみつぶんしりょう: 333.0437715g/mol
- どういたいしつりょう: 333.0437715g/mol
- 同位体原子数: 0
- 水素結合ドナー数: 1
- 水素結合受容体数: 5
- 重原子数: 21
- 回転可能化学結合数: 7
- 複雑さ: 452
- 共有結合ユニット数: 1
- 原子立体中心数の決定: 0
- 不確定原子立体中心数: 0
- 化学結合立体中心数の決定: 0
- 不確定化学結合立体中心数: 0
- 疎水性パラメータ計算基準値(XlogP): 1.5
- トポロジー分子極性表面積: 90.1Ų
benzyl N-{3-2-(chlorosulfonyl)ethyloxetan-3-yl}carbamate 価格詳細 >>
エンタープライズ | No. | 商品名 | Cas No. | 清らかである | 仕様 | 価格 | 更新日時 | 問い合わせ |
---|---|---|---|---|---|---|---|---|
Enamine | EN300-1440620-5000mg |
benzyl N-{3-[2-(chlorosulfonyl)ethyl]oxetan-3-yl}carbamate |
2172460-31-8 | 5000mg |
$6702.0 | 2023-09-29 | ||
Enamine | EN300-1440620-1000mg |
benzyl N-{3-[2-(chlorosulfonyl)ethyl]oxetan-3-yl}carbamate |
2172460-31-8 | 1000mg |
$2311.0 | 2023-09-29 | ||
Enamine | EN300-1440620-50mg |
benzyl N-{3-[2-(chlorosulfonyl)ethyl]oxetan-3-yl}carbamate |
2172460-31-8 | 50mg |
$1942.0 | 2023-09-29 | ||
Enamine | EN300-1440620-500mg |
benzyl N-{3-[2-(chlorosulfonyl)ethyl]oxetan-3-yl}carbamate |
2172460-31-8 | 500mg |
$2219.0 | 2023-09-29 | ||
Enamine | EN300-1440620-10000mg |
benzyl N-{3-[2-(chlorosulfonyl)ethyl]oxetan-3-yl}carbamate |
2172460-31-8 | 10000mg |
$9939.0 | 2023-09-29 | ||
Enamine | EN300-1440620-1.0g |
benzyl N-{3-[2-(chlorosulfonyl)ethyl]oxetan-3-yl}carbamate |
2172460-31-8 | 1g |
$0.0 | 2023-06-06 | ||
Enamine | EN300-1440620-100mg |
benzyl N-{3-[2-(chlorosulfonyl)ethyl]oxetan-3-yl}carbamate |
2172460-31-8 | 100mg |
$2034.0 | 2023-09-29 | ||
Enamine | EN300-1440620-250mg |
benzyl N-{3-[2-(chlorosulfonyl)ethyl]oxetan-3-yl}carbamate |
2172460-31-8 | 250mg |
$2126.0 | 2023-09-29 | ||
Enamine | EN300-1440620-2500mg |
benzyl N-{3-[2-(chlorosulfonyl)ethyl]oxetan-3-yl}carbamate |
2172460-31-8 | 2500mg |
$4530.0 | 2023-09-29 |
benzyl N-{3-2-(chlorosulfonyl)ethyloxetan-3-yl}carbamate 関連文献
-
Xinhua Dai,Zhimin Liu,Buxing Han,Zhenyu Sun,Yong Wang,Jian Xu,Xinglin Guo,Ning Zhao,Jing Chen Chem. Commun., 2004, 2190-2191
-
Hong Bo Wang,Nan Yao,Long Wang,Yu Lin Hu New J. Chem., 2017,41, 10528-10531
-
Monika Kos,Anita Jemec Kokalj,Gordana Glavan,Gregor Marolt,Primož Zidar,Janko Božič,Sara Novak,Damjana Drobne Environ. Sci.: Nano, 2017,4, 2297-2310
-
Wei Liu,Masaru Shiotani,Jacek Michalik,Anders Lund Phys. Chem. Chem. Phys., 2001,3, 3532-3535
-
Julien Lombard,Thierry Biben,Samy Merabia Nanoscale, 2016,8, 14870-14876
2172460-31-8 (benzyl N-{3-2-(chlorosulfonyl)ethyloxetan-3-yl}carbamate) 関連製品
- 1207037-54-4(4-butoxy-N-[1-methyl-5-(morpholine-4-carbonyl)-2-oxo-1,2-dihydropyridin-3-yl]benzamide)
- 69810-77-1(1-(3-methylphenyl)ethane-1,2-diamine)
- 887211-29-2(1-{1-2-(azepan-1-yl)-2-oxoethyl-1H-indol-3-yl}-2-(4-benzylpiperazin-1-yl)ethane-1,2-dione)
- 2138241-73-1(2H-Pyrazolo[4,3-c]pyridine, 3-(6-fluoro-3-pyridinyl)-4,5,6,7-tetrahydro-)
- 953985-48-3(2-(4-fluorophenyl)-N-2-oxo-2-(2-phenylmorpholin-4-yl)ethylacetamide)
- 35155-09-0(4'-(4-Phenylphenoxy)acetophenone)
- 1505799-89-2(3-(2-Methyl-1,3-benzoxazol-4-yl)propanoic acid)
- 930858-73-4(N-(1-cyano-1,2-dimethylpropyl)-2-[4-(pyrimidin-2-yl)piperazin-1-yl]acetamide)
- 660397-15-9(CYCLO(L-ALANYLGLYCYL-L-PROLYL-L-VALYL-L-TYROSYL))
- 158581-29-4(2,4-Dibromo-6-(tert-butyl-NNO-azoxy)phenylamine)
推奨される供給者
BIOOKE MICROELECTRONICS CO.,LTD
ゴールドメンバー
中国のサプライヤー
試薬
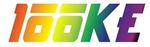
Handan Zechi Trading Co., Ltd
ゴールドメンバー
中国のサプライヤー
大量
Hebei Ganmiao New material Technology Co., LTD
ゴールドメンバー
中国のサプライヤー
大量
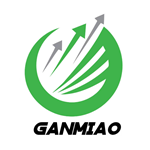
Taizhou Jiayin Chemical Co., Ltd
ゴールドメンバー
中国のサプライヤー
大量
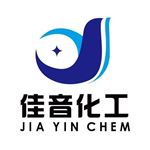
Wuhan brilliant Technology Co.,Ltd
ゴールドメンバー
中国のサプライヤー
大量
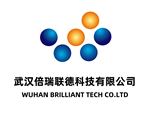