Cas no 1496363-59-7 (5-(4-iodophenyl)-1,3,4-thiadiazol-2-ylmethanamine)
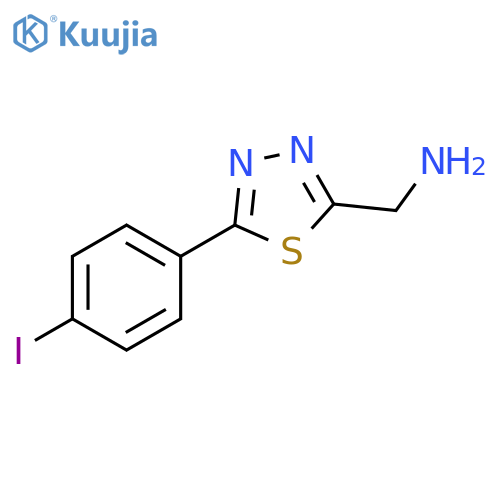
1496363-59-7 structure
商品名:5-(4-iodophenyl)-1,3,4-thiadiazol-2-ylmethanamine
5-(4-iodophenyl)-1,3,4-thiadiazol-2-ylmethanamine 化学的及び物理的性質
名前と識別子
-
- 5-(4-iodophenyl)-1,3,4-thiadiazol-2-ylmethanamine
- 1,3,4-Thiadiazole-2-methanamine, 5-(4-iodophenyl)-
-
- インチ: 1S/C9H8IN3S/c10-7-3-1-6(2-4-7)9-13-12-8(5-11)14-9/h1-4H,5,11H2
- InChIKey: SMCCIIASGDNKKL-UHFFFAOYSA-N
- ほほえんだ: S1C(C2=CC=C(I)C=C2)=NN=C1CN
5-(4-iodophenyl)-1,3,4-thiadiazol-2-ylmethanamine 価格詳細 >>
エンタープライズ | No. | 商品名 | Cas No. | 清らかである | 仕様 | 価格 | 更新日時 | 問い合わせ |
---|---|---|---|---|---|---|---|---|
Enamine | EN300-184197-2.5g |
[5-(4-iodophenyl)-1,3,4-thiadiazol-2-yl]methanamine |
1496363-59-7 | 2.5g |
$1650.0 | 2023-09-19 | ||
Enamine | EN300-184197-0.1g |
[5-(4-iodophenyl)-1,3,4-thiadiazol-2-yl]methanamine |
1496363-59-7 | 0.1g |
$741.0 | 2023-09-19 | ||
Enamine | EN300-184197-10.0g |
[5-(4-iodophenyl)-1,3,4-thiadiazol-2-yl]methanamine |
1496363-59-7 | 10g |
$5405.0 | 2023-05-24 | ||
Enamine | EN300-184197-1g |
[5-(4-iodophenyl)-1,3,4-thiadiazol-2-yl]methanamine |
1496363-59-7 | 1g |
$842.0 | 2023-09-19 | ||
Enamine | EN300-184197-5g |
[5-(4-iodophenyl)-1,3,4-thiadiazol-2-yl]methanamine |
1496363-59-7 | 5g |
$2443.0 | 2023-09-19 | ||
Enamine | EN300-184197-5.0g |
[5-(4-iodophenyl)-1,3,4-thiadiazol-2-yl]methanamine |
1496363-59-7 | 5g |
$3645.0 | 2023-05-24 | ||
Enamine | EN300-184197-0.5g |
[5-(4-iodophenyl)-1,3,4-thiadiazol-2-yl]methanamine |
1496363-59-7 | 0.5g |
$809.0 | 2023-09-19 | ||
Enamine | EN300-184197-1.0g |
[5-(4-iodophenyl)-1,3,4-thiadiazol-2-yl]methanamine |
1496363-59-7 | 1g |
$1256.0 | 2023-05-24 | ||
Enamine | EN300-184197-0.05g |
[5-(4-iodophenyl)-1,3,4-thiadiazol-2-yl]methanamine |
1496363-59-7 | 0.05g |
$707.0 | 2023-09-19 | ||
Enamine | EN300-184197-0.25g |
[5-(4-iodophenyl)-1,3,4-thiadiazol-2-yl]methanamine |
1496363-59-7 | 0.25g |
$774.0 | 2023-09-19 |
5-(4-iodophenyl)-1,3,4-thiadiazol-2-ylmethanamine 関連文献
-
H. Mao,Y. Liao,J. Ma,S. L. Zhao,F. W. Huo Nanoscale, 2016,8, 1049-1054
-
Benny Danilo Belviso,Rocco Caliandro,Dritan Siliqi,Vito Calderone,Fabio Arnesano,Giovanni Natile Chem. Commun., 2013,49, 5492-5494
-
Man Vir Singh,Sudesh Kumar,Moinuddin Sarker Sustainable Energy Fuels, 2018,2, 1057-1068
-
Huy Quang Lê,Yibo Ma,Marc Borrega,Herbert Sixta Green Chem., 2016,18, 5466-5476
1496363-59-7 (5-(4-iodophenyl)-1,3,4-thiadiazol-2-ylmethanamine) 関連製品
- 1227496-85-6(2-2-chloro-5-(trifluoromethyl)pyridin-3-ylacetonitrile)
- 1227270-50-9(5-Bromo-1H-indazol-6-ol)
- 1261830-05-0(2-Hydroxy-6-(trifluoromethoxy)benzylamine)
- 1782249-70-0(2-Thiophenecarboxylic acid, 5-bromo-3-(1-methylethyl)-)
- 1396893-05-2(1-cyclopentyl-3-{5-(2E)-3-phenylprop-2-enoyl-4H,5H,6H,7H-1,3thiazolo5,4-cpyridin-2-yl}urea)
- 921996-19-2(N-(1,3-benzothiazol-2-yl)-2-2-(4-methoxybenzenesulfonamido)-1,3-thiazol-4-ylacetamide)
- 2171464-62-1(4-cyano-2-({(9H-fluoren-9-yl)methoxycarbonyl}amino)-5,5,5-trifluoropentanoic acid)
- 2034293-05-3(1-cyclopentyl-3-(2-methoxyethyl)-1-{2-3-(trifluoromethyl)-1H-pyrazol-1-ylethyl}urea)
- 1261839-48-8(2,5-Difluoro-4-(difluoromethyl)phenol)
- 13769-43-2(potassium metavanadate)
推奨される供給者
Zhejiang Brunova Technology Co., Ltd.
ゴールドメンバー
中国のサプライヤー
大量
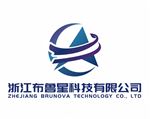
Heyuan Broad Spectrum Biotechnology Co., Ltd
ゴールドメンバー
中国のサプライヤー
試薬
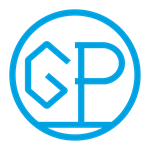
Zouping Mingyuan Import and Export Trading Co., Ltd
ゴールドメンバー
中国のサプライヤー
試薬
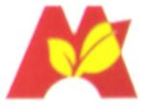
Suzhou Genelee Bio-Technology Co., Ltd.
ゴールドメンバー
中国のサプライヤー
大量
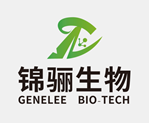
Jiangxi Boyang Pharmaceutical Chemical Co., Ltd
ゴールドメンバー
中国のサプライヤー
大量
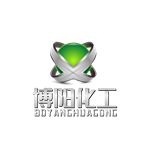