Cas no 132843-44-8 (Lithium bis(pentafluoroethanesulfonyl)imide)
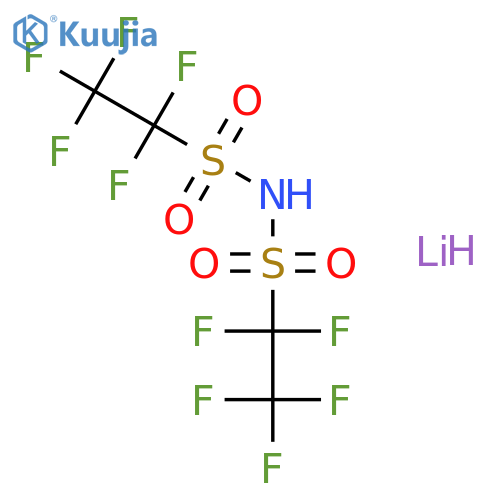
132843-44-8 structure
商品名:Lithium bis(pentafluoroethanesulfonyl)imide
CAS番号:132843-44-8
MF:C4F10LiNO4S2
メガワット:387.102133750916
MDL:MFCD22374096
CID:1231167
Lithium bis(pentafluoroethanesulfonyl)imide 化学的及び物理的性質
名前と識別子
-
- lithium 1,1,2,2,2-pentafluoro-N-(1,1,2,2,2-pentafluoroethylsulfonyl)ethanesulfonamide
- ethanesul<wbr>
- LogP
- Lithium Bis(pentafluoroethanesulfonyl)imide
- lithium,bis(1,1,2,2,2-pentafluoroethylsulfonyl)azanide
- Bis(pentafluoroethanesulfonyl)imide Lithium Salt
- LiBETI
- Lithium bis(pentafluoroethanesulfonyl)amide
- Ethanesulfonamide, 1,1,2,2,2-pentafluoro-N-[(pentafluoroethyl)sulfonyl]-, lithium salt
- lithium bis(perfluoroethanesulfonyl)imide
- lithium bis(pentafluoroethylsulfonyl)imide
- L0267
- Ethanesulfonamide, 1,1,2,2,2-pentafluoro-N-[(1,1,2,2,2-pentafluoroethyl)sulfonyl]-, lithium salt (1:1)
- Lithium bis(pentafluoroethanesulfonyl)imide
-
- MDL: MFCD22374096
- インチ: 1S/C4F10NO4S2.Li/c5-1(6,7)3(11,12)20(16,17)15-21(18,19)4(13,14)2(8,9)10;/q-1;+1
- InChIKey: ACFSQHQYDZIPRL-UHFFFAOYSA-N
- ほほえんだ: S(C(C(F)(F)F)(F)F)([N-]S(C(C(F)(F)F)(F)F)(=O)=O)(=O)=O.[Li+]
計算された属性
- せいみつぶんしりょう: 386.93385
- 水素結合ドナー数: 0
- 水素結合受容体数: 15
- 重原子数: 22
- 回転可能化学結合数: 4
- 複雑さ: 539
- トポロジー分子極性表面積: 86
じっけんとくせい
- ゆうかいてん: 328°C(lit.)
- PSA: 80.31
Lithium bis(pentafluoroethanesulfonyl)imide セキュリティ情報
-
記号:
- ヒント:あぶない
- 危害声明: H301+H311-H314-H412
- 警告文: P260-P264-P270-P273-P280-P301+P330+P331+P310-P303+P361+P353+P310+P363-P304+P340+P310-P305+P351+P338+P310-P405-P501
- 危険物輸送番号:2923
- 危険レベル:8/6.1
- 包装グループ:III
Lithium bis(pentafluoroethanesulfonyl)imide 税関データ
- 税関コード:29319090
Lithium bis(pentafluoroethanesulfonyl)imide 価格詳細 >>
エンタープライズ | No. | 商品名 | Cas No. | 清らかである | 仕様 | 価格 | 更新日時 | 問い合わせ |
---|---|---|---|---|---|---|---|---|
TI XI AI ( SHANG HAI ) HUA CHENG GONG YE FA ZHAN Co., Ltd. | L0267-1G |
Lithium Bis(pentafluoroethanesulfonyl)imide |
132843-44-8 | >98.0%(T) | 1g |
¥695.00 | 2024-04-17 | |
abcr | AB357949-25 g |
Lithium bis(pentafluoroethanesulfonyl)imide; . |
132843-44-8 | 25 g |
€993.60 | 2023-07-19 | ||
TRC | L469393-1g |
Lithium Bis(pentafluoroethanesulfonyl)imide |
132843-44-8 | 1g |
200.00 | 2021-08-04 | ||
abcr | AB357949-10 g |
Lithium bis(pentafluoroethanesulfonyl)imide; . |
132843-44-8 | 10g |
€238.00 | 2022-08-31 | ||
eNovation Chemicals LLC | Y1054629-1g |
Lithium Bis(pentafluoroethanesulfonyl)imide |
132843-44-8 | 98.0% | 1g |
$185 | 2023-05-17 | |
SHANG HAI MAI KE LIN SHENG HUA Technology Co., Ltd. | L849464-250mg |
Lithium Bis(pentafluoroethanesulfonyl)imide |
132843-44-8 | 98%,(T) | 250mg |
¥248.00 | 2022-01-10 | |
SHANG HAI MAI KE LIN SHENG HUA Technology Co., Ltd. | L849464-1g |
Lithium Bis(pentafluoroethanesulfonyl)imide |
132843-44-8 | 98%,(T) | 1g |
¥960.00 | 2022-01-10 | |
SHENG KE LU SI SHENG WU JI SHU | sc-472244-5 g |
Lithium bis(perfluoroethylsulfonyl)imide, |
132843-44-8 | 5g |
¥3,384.00 | 2023-07-11 | ||
1PlusChem | 1P009H3C-5g |
Lithium Bis(pentafluoroethanesulfonyl)imide |
132843-44-8 | >98.0%(T) | 5g |
$192.00 | 2025-02-24 | |
Ambeed | A763653-250mg |
LIthium bis(pentafluoroethanesulfonyl)imide |
132843-44-8 | 98% | 250mg |
$44.0 | 2024-04-24 |
Lithium bis(pentafluoroethanesulfonyl)imide 関連文献
-
Bo Xu,Qing-Shan Liang,Lian-Tao Liu,Qi-Sheng Liu,Cun-Cheng Li RSC Adv., 2014,4, 13919-13926
-
Anton Ivanov,Sebastian Boldt,Zaib un Nisa,Syed Jawad Ali Shah,Alexander Villinger,Gyula Schneider,János Wölfling,Jamshed Iqbal RSC Adv., 2016,6, 11118-11127
-
Zhentan Lu,Lin Mei,Xinge Zhang,Yanan Wang,Yu Zhao,Chaoxing Li Polym. Chem., 2013,4, 5743-5750
-
Kelsey M. Hallinen,Stephanie Tristram-Nagle,John F. Nagle Phys. Chem. Chem. Phys., 2012,14, 15452-15457
-
5. Water
132843-44-8 (Lithium bis(pentafluoroethanesulfonyl)imide) 関連製品
- 2092798-12-2(4-(3,4-Difluorophenyl)-3,3-dimethylpyrrolidine)
- 866845-59-2(N-(4-chlorophenyl)-5-methyl-1-(3-methylphenyl)-1H-1,2,3-triazole-4-carboxamide)
- 30764-59-1((4-ethylphenyl)-(furan-2-yl)methanone)
- 1804850-80-3(4-(Aminomethyl)-3-(difluoromethyl)-6-(trifluoromethoxy)-2-(trifluoromethyl)pyridine)
- 26406-97-3(4-Amino-3-chloro-hydratropic Acid Ethyl Ester)
- 500780-11-0(α-Phenyl-2-piperidineacetonitrile)
- 1806300-01-5(1-(2-Ethoxybenzo[d]oxazol-4-yl)ethanone)
- 1267109-65-8(5-Thiazoleacetic acid, 2-(aminomethyl)-4-methyl-)
- 1250552-15-8(N-cyclooctyl-1-methylpyrrolidin-3-amine)
- 1572134-89-4(2-Amino-1-(4-(pyridin-4-yl)piperazin-1-yl)propan-1-one)
推奨される供給者
Amadis Chemical Company Limited
(CAS:132843-44-8)Lithium bis(pentafluoroethanesulfonyl)imide
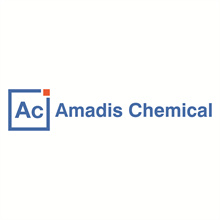
清らかである:99%
はかる:25g
価格 ($):589.0