Cas no 101996-62-7 (Citric Acid 1,3-Dibenzylester)
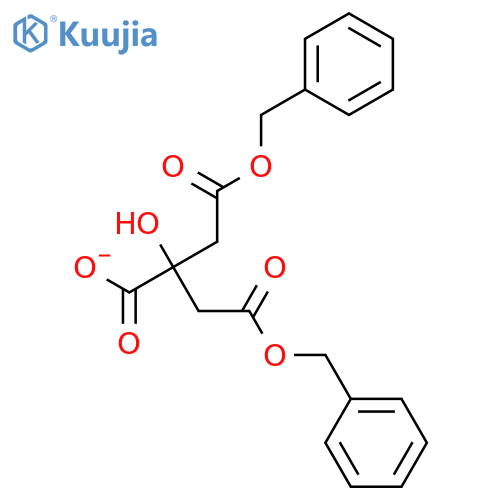
Citric Acid 1,3-Dibenzylester structure
商品名:Citric Acid 1,3-Dibenzylester
CAS番号:101996-62-7
MF:C20H20O7
メガワット:372.368606567383
CID:1131439
Citric Acid 1,3-Dibenzylester 化学的及び物理的性質
名前と識別子
-
- 1,2,3-Propanetricarboxylic acid, 2-hydroxy-, 1,3-bis(phenylmethyl) ester
- Citric Acid 1,3-Dibenzylester
-
計算された属性
- せいみつぶんしりょう: 371.11307
じっけんとくせい
- PSA: 112.96
Citric Acid 1,3-Dibenzylester 価格詳細 >>
エンタープライズ | No. | 商品名 | Cas No. | 清らかである | 仕様 | 価格 | 更新日時 | 問い合わせ |
---|---|---|---|---|---|---|---|---|
TRC | C521015-50mg |
Citric Acid 1,3-Dibenzylester |
101996-62-7 | 50mg |
$270.00 | 2023-05-18 | ||
TRC | C521015-100mg |
Citric Acid 1,3-Dibenzylester |
101996-62-7 | 100mg |
$500.00 | 2023-05-18 | ||
TRC | C521015-250mg |
Citric Acid 1,3-Dibenzylester |
101996-62-7 | 250mg |
$1206.00 | 2023-05-18 | ||
TRC | C521015-500mg |
Citric Acid 1,3-Dibenzylester |
101996-62-7 | 500mg |
$2113.00 | 2023-05-18 | ||
TRC | C521015-1g |
Citric Acid 1,3-Dibenzylester |
101996-62-7 | 1g |
$ 3000.00 | 2023-09-08 |
Citric Acid 1,3-Dibenzylester 関連文献
-
Ibrar Ayub,Dangsheng Su,Marc Willinger,Alexey Kharlamov,Leonid Ushkalov,Valery A. Zazhigalov,Nataly Kirillova,Robert Schlögl Phys. Chem. Chem. Phys., 2003,5, 970-978
-
Yue Liu,Qian Zhao,Xiaohong Wang,Shiwu Li RSC Adv., 2013,3, 13748-13755
-
Patrick Johannes Riss,René Hummerich,Patrick Schloss Org. Biomol. Chem., 2009,7, 2688-2698
-
Sohyun Park,Hungu Kang,Hyo Jae Yoon J. Mater. Chem. A, 2019,7, 14419-14446
-
5. Translating endoplasmic reticulum biology into the clinic: a role for ER-targeted natural products?David M. Pereira,Patrícia Valentão,Georgina Correia-da-Silva,Natércia Teixeira,Paula B. Andrade Nat. Prod. Rep., 2015,32, 705-722
101996-62-7 (Citric Acid 1,3-Dibenzylester) 関連製品
- 952068-64-3(Parishin D)
- 1091618-41-5(5-(4-Bromo-2-methoxyphenyl)oxazole)
- 1218591-94-6(2-[3-(hydroxymethyl)piperidin-1-yl]propanoic acid)
- 1021228-59-0(N-(3,5-dimethylphenyl)-2-{[5-(3,4-dimethylphenyl)-1,3-oxazol-2-yl]sulfanyl}acetamide)
- 1805747-85-6(4-(2-Bromopropanoyl)-2-methylphenylacetic acid)
- 1805870-06-7(Methyl 4-(2-carboxyethyl)-2-cyanobenzoate)
- 2092798-12-2(4-(3,4-Difluorophenyl)-3,3-dimethylpyrrolidine)
- 1481342-96-4(3-amino-1-(pyridin-4-yl)propan-1-one)
- 1342842-36-7(3,4,5-trifluoro-N-(2-methylbutyl)aniline)
- 2136903-86-9((3S)-3-{(benzyloxy)carbonylamino}-3-(5-chloro-1-methyl-1H-pyrazol-4-yl)propanoic acid)
推奨される供給者
Shanghai Joy Biotech Ltd
ゴールドメンバー
中国のサプライヤー
大量
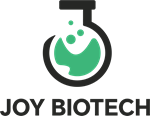
Zhangzhou Sinobioway Peptide Co.,Ltd.
ゴールドメンバー
中国のサプライヤー
試薬
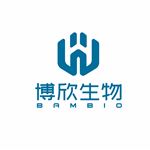
Chong Da Prostaglandin Fine Chemicals Co., Ltd.
ゴールドメンバー
中国のサプライヤー
試薬
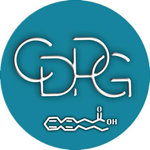
Heyuan Broad Spectrum Biotechnology Co., Ltd
ゴールドメンバー
中国のサプライヤー
試薬
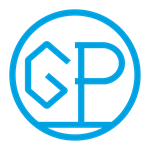
上海帛亦医药科技有限公司
ゴールドメンバー
中国のサプライヤー
試薬