Cas no 1002750-88-0 (O-(5-chloropyridin-2-yl)methylhydroxylamine)
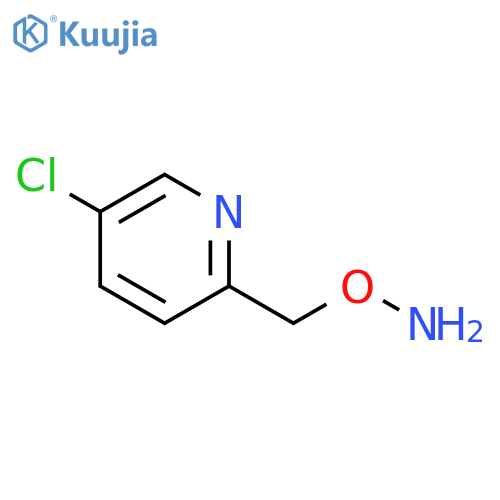
1002750-88-0 structure
商品名:O-(5-chloropyridin-2-yl)methylhydroxylamine
CAS番号:1002750-88-0
MF:C6H7ClN2O
メガワット:158.58558011055
MDL:MFCD28675662
CID:5229721
PubChem ID:57993056
O-(5-chloropyridin-2-yl)methylhydroxylamine 化学的及び物理的性質
名前と識別子
-
- Hydroxylamine, O-[(5-chloro-2-pyridinyl)methyl]-
- O-(5-chloropyridin-2-yl)methylhydroxylamine
-
- MDL: MFCD28675662
- インチ: 1S/C6H7ClN2O/c7-5-1-2-6(4-10-8)9-3-5/h1-3H,4,8H2
- InChIKey: LYEJLKVVLRTBPG-UHFFFAOYSA-N
- ほほえんだ: NOCC1=NC=C(Cl)C=C1
O-(5-chloropyridin-2-yl)methylhydroxylamine 価格詳細 >>
エンタープライズ | No. | 商品名 | Cas No. | 清らかである | 仕様 | 価格 | 更新日時 | 問い合わせ |
---|---|---|---|---|---|---|---|---|
eNovation Chemicals LLC | Y1195616-1g |
O-[(5-Chloro-2-pyridyl)methyl]hydroxylamine |
1002750-88-0 | 95% | 1g |
$965 | 2025-02-21 | |
Enamine | EN300-1986712-0.5g |
O-[(5-chloropyridin-2-yl)methyl]hydroxylamine |
1002750-88-0 | 0.5g |
$809.0 | 2023-09-16 | ||
Enamine | EN300-1986712-10.0g |
O-[(5-chloropyridin-2-yl)methyl]hydroxylamine |
1002750-88-0 | 10g |
$4729.0 | 2023-06-02 | ||
Enamine | EN300-1986712-0.25g |
O-[(5-chloropyridin-2-yl)methyl]hydroxylamine |
1002750-88-0 | 0.25g |
$774.0 | 2023-09-16 | ||
Enamine | EN300-1986712-5.0g |
O-[(5-chloropyridin-2-yl)methyl]hydroxylamine |
1002750-88-0 | 5g |
$3189.0 | 2023-06-02 | ||
Enamine | EN300-1986712-0.1g |
O-[(5-chloropyridin-2-yl)methyl]hydroxylamine |
1002750-88-0 | 0.1g |
$741.0 | 2023-09-16 | ||
eNovation Chemicals LLC | Y1195616-1g |
O-[(5-Chloro-2-pyridyl)methyl]hydroxylamine |
1002750-88-0 | 95% | 1g |
$965 | 2024-07-19 | |
Enamine | EN300-1986712-2.5g |
O-[(5-chloropyridin-2-yl)methyl]hydroxylamine |
1002750-88-0 | 2.5g |
$1650.0 | 2023-09-16 | ||
Enamine | EN300-1986712-0.05g |
O-[(5-chloropyridin-2-yl)methyl]hydroxylamine |
1002750-88-0 | 0.05g |
$707.0 | 2023-09-16 | ||
Enamine | EN300-1986712-1.0g |
O-[(5-chloropyridin-2-yl)methyl]hydroxylamine |
1002750-88-0 | 1g |
$1100.0 | 2023-06-02 |
O-(5-chloropyridin-2-yl)methylhydroxylamine 関連文献
-
Yi-Tao Xu,Joyce Li Nanoscale Horiz., 2022,7, 185-191
-
Miguel A. C. Teixeira,Steve Arscott,Simon J. Cox Soft Matter, 2018,14, 5369-5382
-
Juan David Serna-Salazar,Jorge Mahecha-Gómez Phys. Chem. Chem. Phys., 2000,2, 4061-4065
-
Vyacheslav Y. Sosnovskikh,Alexander V. Safrygin,Marina A. Ezhikova RSC Adv., 2016,6, 30056-30069
-
Wei-Min Ren,Meng-Wei Liang,Yue-Chao Xu,Xiao-Bing Lu Polym. Chem., 2013,4, 4425-4433
1002750-88-0 (O-(5-chloropyridin-2-yl)methylhydroxylamine) 関連製品
- 2172527-63-6(4-1-(hydroxymethyl)-3,3-dimethylcyclobutyl-1-methylpiperidin-4-ol)
- 2680735-87-7(tert-butyl N-(5-nitro-1,3-benzoxazol-2-yl)carbamate)
- 68551-17-7(Isoalkanes, C10-13)
- 2138063-83-7(2-(3-methoxy-1,2-thiazol-4-yl)-2-methylpropanoic acid)
- 941902-66-5(1-(3-fluorophenyl)methyl-3-(pyridin-4-yl)methyl-1H,2H,3H,4H-pyrido3,2-dpyrimidine-2,4-dione)
- 1171210-43-7(N-[2-(3,5-dimethyl-1H-pyrazol-1-yl)ethyl]-N-(4-ethoxy-1,3-benzothiazol-2-yl)cyclopropanecarboxamide)
- 2227651-43-4(rac-(1R,3R)-3-(3-fluoro-4-methylphenyl)-2,2-dimethylcyclopropylmethanamine)
- 2034569-13-4(N-2-(1-benzothiophen-3-yl)-2-(dimethylamino)ethyl-3,4-difluorobenzamide)
- 102408-28-6(1-Methyl-2-methylaminoimidazo4,5-Fquinoline)
- 99094-20-9(4,4'-(1,2-Diphenyl-1,2-ethenediyl)dianiline)
推奨される供給者
钜澜化工科技(青岛)有限公司
ゴールドメンバー
中国のサプライヤー
大量
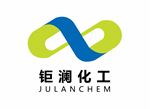
Hubei Changfu Chemical Co., Ltd.
ゴールドメンバー
中国のサプライヤー
大量
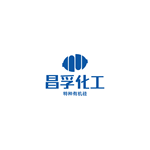
Jinan Hanyu Chemical Co.,Ltd.
ゴールドメンバー
中国のサプライヤー
大量
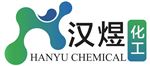
Jinta Yudi Pharmaceutical Technology Co., Ltd.
ゴールドメンバー
中国のサプライヤー
大量
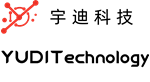
Hubei Henglvyuan Technology Co., Ltd
ゴールドメンバー
中国のサプライヤー
大量